Translate this page into:
Human dental pulp stem cells and its applications in regenerative medicine – A literature review
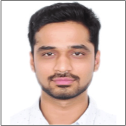
*Corresponding author: Dr. Saravana Priyan GL, Balaji Dental and Craniofacial Hospital, No.30, KB Dasan Road, Teynampet, Chennai- 600 018, Tamil Nadu, India. drsaravanapriyangl@gmail.com
-
Received: ,
Accepted: ,
How to cite this article: Saravana Priyan GL, Ramalingam S, Udhayakumar Y. Human dental pulp stem cells and its applications in regenerative medicine – A literature review. J Global Oral Health 2019;2(1):59-67.
Abstract
Human dental pulp-derived stem cells have varied applications in regenerative medicine. Dental pulp stem cells (DPSCs) are considered to be neural crest cells. They are known to have higher regenerative potential than the bone marrow-derived mesenchymal stem cells. DPSCs have multipotency, immunomodulatory function, and self-renewal capacity. They are highly proliferative, clonogenic and are capable of differentiating into adipocytes, neural cells, odontoblasts, and various other cells. DPSCs are effective for various diseases, such as spinal cord injuries, Parkinson’s disease, Alzheimer’s disease, cerebral ischemia, myocardial infarction, muscular dystrophy, diabetes, liver diseases, eye diseases, immune diseases, and oral diseases. This article provides an overview of properties and regenerative applications of human DPSCs.
Keywords
Human dental pulp-derived stem cells
Dental pulp stem cells neural crest cells
Regenerative medicine
INTRODUCTION
Stem cells are clonogenic cells capable of self-renewal and multi-lineage differentiation. Post- natal stem cells/adult stem cells were first isolated from bone marrow. They were later isolated from the neural tissue, retina, and even the skin.[1] The bone marrow-derived stem cells are most widely researched and utilized in clinical settings.
Dental pulp stem cells (DPSC) were first discovered in the year 2000, from an extracted impacted third molar by Gronthos et al.[2] DPSCs are considered to be cranial neural crest cells (CNCCs). A group of NCCs migrate from the neural crest and is temporally formed between ectoderm and neural plate during neural tube formation. They play an important role in embryo development. During the migration, the NCCs translate into mesenchymal cells.
The CNCCs concentrate in facial and pharyngeal arches they form sensory VII, IX, X cranial nerves, thymus, thyroid follicular cells, parathyroid, and cornea. They also form the orofacial mesenchymal organs including facial skeleton such as maxilla, mandible, dentin/pulp complex, cementum, periodontal ligament (PDL), and alveolar bone.[3]
The natural function of DPSCs in the production of odontoblasts to create reparative dentin aids in the regeneration of tooth structures. However, they are also effective in the repair of tissues outside the tooth. The ease of isolation of DPSCs from discarded or removed teeth offers a promising source of autologous cells, and their similarities with bone marrow stromal cells (BMSCs) suggest applications in musculoskeletal regenerative medicine.[4]
DPSCs are effective for various diseases, such as spinal cord injuries (SCIs), Parkinson’s disease (PDs), Alzheimer’s disease, cerebral ischemia, myocardial infarction, muscular dystrophy, diabetes, liver diseases, eye diseases, immune diseases, and oral diseases.[5]
Other types of human dental pulp-derived stem cells (HDPSCs) include dental pulp of human exfoliated deciduous teeth, root apical papilla of human teeth, and dental pulp of human supernumerary teeth, namely, stem cells from human exfoliated deciduous teeth (SHED), stem cells from apical papilla (SCAP), and human supernumerary tooth-derived stem cells (SNTSCs) were identified in the year 2003, 2006, and 2013 retrospectively [Figure 1]. In addition to this, stem cells can be isolated from various tissues, including oral parts such as alveolar bone, PDL, dental follicle, oral mucosa, and gingival.

- Types of human dental pulp derived stem cells.
Recently, cryopreservation of human cells and tissues is proving to be a reliable and feasible approach for stem cell storage.[6]
PROPERTIES OF HUMAN DENTAL PULP- DERIVED STEM CELLS
The HDPSCs share the common properties of mesenchymal stem cells. They have undifferentiated lineage with long-term self-renewal capacity. They also have the ability to develop into progenitor cells. They can differentiate into mesodermal, ectodermal, endodermal, osteogenic, chondrogenic, and adipogenic lineages.[7]
The properties of HDPSCs include:
Multipotency
Stem cell technology enables to induce HDPSCs into ectodermal lineage cells such as neural cells;[2,8] mesenchymal lineage cells such as odontoblasts, osteoblasts, chondrocytes, adipocytes, and mycocytes;[9] endodermal lineage cells such as vascular endothelial cells,[9] hepatocytes,[10] and pancreatic islet-insulin-producing β cells[11] [Figure 2].

- Multilineage differentiation of human dental pup-derived stem cells.
High proliferation activity
Population doubling assay shows HDPSCs express higher proliferative ability (3–4 times higher) than human bone marrow-derived mesenchymal stem cells (BMMSCs).[2,8-13] In addition, HDPSCs also express higher telomerase activities.[12,14]
Self-renewal capacity
HDPSCs represent a novel adult stem cell that possesses high proliferative potential with long-term self-renewal capacity.[1,2,8-13]
In vivo tissue regeneration capacity
When HDPSCs are subcutaneously transplanted with hydroxyapatite/tricalcium phosphate (HA/TCP) powders as carrier, into the dorsal surface of immune compromised mice, individual HDPSCs expressed a specific and unique regeneration capability.[2,8-13] DPSCs and SCAP not only regenerate dentin[2,13] but are also able to induce dental-pulp- like tissues containing blood capillary vessels and dense collagen fibers surrounded by the newly formed dentin. Thus, DPSCs and SCAP can reconstruct de novo dentin/pulp complex in vivo, thereby proving that DPSCs and SCAP are effective cell sources to regenerate dentin/pulp complex structures.
SHED and SNTSCs express a unique in vivo bi-potency. In vivo transplantation of SHED and SNTSCs with HA/TCP not only form dentin/pulp complex-like structures but also reconstruct bone/bone marrow units. These findings suggest that they might consider a unique cell source to regenerated dentin/pulp complex and bone/bone marrow unit.[8,12]
Colony-forming unit-fibroblasts forming ability
HDPSCs form adherent colonies that consist of spindle- shaped cells, called colony-forming unit-fibroblasts (CFU-F). Amazingly, CFU-F analysis shows that human dental pulp contains abundant MSCs than human bone marrow stem cells. (CFU-F capacity of DPSCs is 5 times higher than human bone marrow-derived MSCs).[2,8-13]
Expression of cell-surface markers
HDPSCs express negative to hematopoietic cell-surface markers including CD34, CD45, and CD14. On the other hand, dental pulp-derived stem cells express positive to STRO-1, CD146 (melanoma cell adhesion molecule), CD105 (endoglin or SH2), and CD73 (5’-nucleotidase [5’-NT] or SH3/4), as well as CD90 (Thy-1) and CD29 (integrin beta-1).[2,8-13] These markers are known as specific markers for MSCs. In addition, HDPSCs express not only markers of embryonic stem cells, stage-specific embryonic antigen-4, Nanog, and Octamer 4, but also markers of NCCs, Nestin, Notch1, and CD271 (p71 neuritrophin receptor or low-affinity nerve growth factor receptor).[12,14] Interestingly, CD24 is expressed only on SCAP among four types of HDPSCs.[13]
Immunomodulation
HDPSCs can affect the immune cells such as T cells directly or indirectly.[15,16] HDPSCs are able to inhibit the proliferation of T cells, downregulate proinflammatory interleukin (IL) 17-secreting helper T cells, and upregulate regulatory T cells.[14,17,18] HDPSCs regulate T cell proliferation through releasing of transforming growth factor-β1 (TGF-β), hepatocyte growth factor, and indoleamine 2, 3-dioxygenase (IDO).[15] HDPSCs express Fas ligand to induce apoptosis of T-cells.[17,18]
APPLICATIONS OF HUMAN DENTAL PULP- DERIVED STEM CELLS
The various applications of HDPSCs based on recent studies with respect to tissue regenerative capacity, multipotency, and immunomodulatory factors [Figure 3].

- Various applications of human dental pulp-derived stem cells.
Tissue engineering
Regeneration of dentin/pulp complex
The regeneration of dentin pulp complex is based on vascularization. Vascular endothelial growth factor administration promotes vascularization but has a short half-life. This can be increased by binding to heparin.[19] Treating stem cells under hypoxic conditions induce the cells to secrete vascularizing agents.[20] Stem cells differentiate into various types of cells; hence, they have to be controlled using growth factors like soluble protein of the dentin matrix. DPSCs were mixed with a carrier and filled in a root canal treated extracted tooth, and the DPSC filled tooth was transplanted into dorsal surface of immune-compromised mice. Regenerated dentin deposited along to existing dentin and connective tissues beneath the de novo dentin contains blood vessels.[21]
Autologous transplantation of DPSCs is clinically tried to regenerate the dentin-pulp complex. Tubular dentin formation was observed when human pulp stem cells with scaffold (HA/tricalcium phosphate) were implanted in immunocompromised mice.[1] Reparative dentin formation on amputated pulp was found when stem cells were combined with recombinant human bone morphogenetic protein 2 in experimental studies on animal models.[22]
Periodontal regeneration
Kawaguchi et al. used BMSCs for their ability to produce alveolar bone, PDL, and in vivo cementum after implantation into the periodontal defects thereby proving to be an alternative source in the treatment of periodontal diseases.[23,24] Marei et al. in their experiment on goat was able to regenerate periodontal tissues around titanium implant using autologous bone marrow stem cells with the scaffold.[25] Transplantation of PDL derived cells into animal models was shown to regenerate periodontal tissue.[26]
Iwata et al. harvested and expanded primary canine PDL cells in vitro and also made into transplantable constructs containing PGA scaffold and PDL cell sheets. The transplantable constructs in combination with porous b-tricalcium phosphate induced regeneration of periodontal structures, including alveolar bone, cementum, and periodontal fibers.[27]
Liu et al. regenerated periodontal tissue in miniature swine using scaffolds seeded with PDL derived stem cells (PDLSCs).[28] PDLSCs can differentiate into cells that can colonize on the biocompatible scaffold, suggesting an easy and efficient autologous source of stem cells for regeneration of dental tissues.[29]
Root regeneration
SCAP has remarkable cell migration activity; which is considered to involve root growth in tooth development. When SCAP-immersed root-formed HA/TCP carriers were subcutaneously grafted into the dorsal surface of immune- compromised mice, dentin/pulp-complex-like structure is formed in the root-formed carrier. In addition, when a root formed carrier containing SCAPs covered with PDLSC- immersed absorbable gelatin sponge is implanted into a socket of the mandibular bone of a swine, the root-form carrier is reconstructed with newly formed dentin/pulp-complex and is surrounded by regenerated PDL on de novo cementum. The regenerated tooth root-like structure works functionally as a masticatory organ likely natural porcine teeth.[10]
Bone regeneration
TH [4-(4-methoxyphenyl)pyrido[40,30:4,5]thieno[2,3-b] pyridine-2-carboxamide], a helioxanthin derivative, induces osteogenic differentiation of preosteoblastic and mesenchymal cells[30] in vitro and in vivo,[31-33] and the optimal concentration for producing the osteogenic effects of TH on MC3T3-E1 and C3H10T1/2 cell lines is 10–6 M.[33]
d’Aquino et al. evaluated bone regeneration by DPSCs both clinically and radiographically, using a collagen scaffold. Their results showed that within 3 months of colonization on the scaffold, complete radiographic bone regeneration could be observed.[34] de Mendonça Costa et al. evaluated the capacity of human DPSCs to reconstruct large cranial defects in non- immunosuppressed rats and found that a more mature bone was formed in the cranial defects, supplied with collagen membrane and HDPSCs.[35] Chadipiralla et al. studied the osteogenic differentiation of stem cells derived from human PDLs and the pulp of human exfoliated deciduous teeth and suggested that PDLSC is a better osteogenic stem cell source.[36]
Lymper et al. suggested that positioning of a biocomplex of collagen sponge filled with DPSCs in the extracted site of mandibular third molar resulted in a higher rate of mineralization and cortical levels leading to complete regeneration. The samples also showed a well-organized and vascularized bone with a lamellar architecture surrounding the Haversian canal was observed.[37] They also prove to be a useful tool for the treatment of degenerative diseases involving the maxilla and mandible.[37]
Tooth regeneration
Duailibi et al., in their experimental studies, were able to form tooth structures from single-cell suspensions of cultured rat tooth bud cells. They demonstrated bioengineered rat teeth developed in 12 weeks with PGA and PLGA scaffold.[38] Honda et al. developed tissue-engineered teeth when implanted into omentum of the rat using porcine tooth bud cells and PGA fiber mesh scaffold resembling odontogenesis. Histological analysis showed that the pattern of tissue-engineered odontogenesis was similar to that of natural tooth development with significant regeneration of enamel, dentin, and cementum.[39]
Cell transplantation
The efficiency of cell transplantation of HDPSCs in various diseases is discussed.
Central nervous system
The CNS typically has a poor ability to repair and regenerate new neurons because of its limited pool of precursor cells.[40] Exogenous stem cells (DPSCs) will lead to both regeneration of new neural precursor cells and their enhanced neuronal and glial differentiation. They will also lead to survival and maintenance of existing neural cells through secretion of trophic factors.[41,42]
SCI
SCI involves an initial primary tissue disruption (e.g., mechanical damage to nerve cells and blood vessels) and then a secondary injury caused by neuroinflammatory responses (e.g., excitotoxicity, blood-brain barrier disruption, oxidative stress, and apoptosis).[43] DPSCs differentiating into neuron-like and oligodendrocyte-like cells that may promote axonal regeneration and tissue repair after SCI.[44-46] DPSCs also reduce secondary inflammatory injury, which facilitates axonal regeneration and reduces progressive hemorrhagic necrosis associated with IL-1β, ras homolog gene family member A, and sulfonylurea receptor 1 expression.[47] DPSCs when transplanted together with artificial scaffolds like chitosan promotes motor functional recovery and inhibits cell apoptosis after SCI by secreting BDNF, GDNF, and NT-3 and reducing the expression of active-caspase 3.[48,49]
Stroke
Stroke is an ischemic cerebrovascular condition that leads to brain damage, long-term disability, and even death. DPSCs promote functional recovery after ischemic stroke by immunomodulation. Some in vivo studies have shown that transplantation of DPSCs into the ischemic areas of middle cerebral artery occlusion in Sprague-Dawley rats promoted locomotor functional recovery and decreased infarct areas by their differentiation into dopaminergic (DA) neurons and secretion of neurotrophic factors.[50,51] DPSC transplantation into ischemic areas of focal cerebral ischemia in rats led to the expression of proangiogenic factors that supported dense capillary formation and renormalization of blood flow.[52] Intracerebral transplantation of DPSCs into regions of focal cerebral ischemia in rodent models promoted forelimb sensory and motor functional recovery at 4 weeks post-treatment.[53] DPSCs also provided cytoprotection for astrocytes by reducing reactive gliosis and preventing free radical and IL-1β secretion within in vitro ischemic models.[54]
Parkinson’s
PDs is a progressive neurodegenerative condition associated with loss of nigrostriatal DA neurons leading to muscle rigidity, bradykinesia, postural instability, and resting tremor.[55] Intrathecal transplantation of DPSCs into the 1-methyl-4- phenyl-1,2,3,6-tetrahydropyridine (MPTP) induced old-aged mouse model of PD, promoted recovery of behavioral deficits, restored DA functions, and attenuated MPTP-induced damage by reducing the secretion of proinflammatory factors such as IL-1α, IL-1β, IL6, IL8, and tumor necrosis factor (TNF)-α and by upregulating the expression levels of anti- inflammatory factors such as IL2, IL4, and TNF-β.[56] DPSCs also showed neuroimmunomodulatory activity in an in vitro model of PD by reducing MPTP-induced deficits associated with reactive oxygen species, DNA damage, and nitric oxide release.[57]
Alzheimer’s
Alzheimer’s disease (AD) is a progressive neurodegenerative condition caused by the loss of neurons, intracellular neurofibrillary tangles, and deposition of insoluble β-amyloid peptides in the brain.[58] Clinical symptoms of AD include memory loss, cognitive deficits, and linguistic disorders.[59] DPSCs promoted neuronal repair and regeneration by restoring cytoskeletal structure, protecting microtubule stability, and reducing tau phosphorylation in the okadaic acid-induced cellular model of AD.[60] DPSCs can also reduce amyloid-beta (Aβ) peptide-induced cytotoxicity and apoptosis in the AD cellular model by secreting higher levels of VEGF, fractalkine, RANTES, fms-related tyrosine kinase 3, and monocyte chemotactic protein 1.[61,62]
Retinal injury
The retina is a part of the CNS and is composed of photoreceptors, bipolar cells, and retinal ganglion cells (RGCs).[63] Head injuries can cause traumatic optic neuropathy while ocular chronic degenerative diseases such as glaucoma lead to the slow loss of RGCs.[64] DPSC transplantation into the vitreous of optic nerve injury rat model could promote axonal regeneration and RGC survival by a neurotrophin-mediated mechanism.[65] Intravitreal transplantation of DPSCs in an animal model of glaucoma maintained visual function up to 35 days after treatment by preventing RGC death.[66]
Peripheral nerve injury
Peripheral nerve injury caused by traumatic accidents and iatrogenic damage often accompanies physical disability and neuropathic pain. Autologous nerve grafting is the preferred treatment choice for a long gap of peripheral nerve deficits. Studies suggest that DPSC-embedded biomaterial nerve conduits such as polylactic glycolic acid tubes have the ability to promote regeneration of injured facial nerve and to improve functional recovery comparable to that of autografts.[67] Collagen conduits loaded with Schwann-like cells induced from DPSCs in vitro have facilitated repair and regeneration of 15 mm sciatic nerve defect.[68]
Autoimmune diseases
The major mechanisms may involve the secretion of soluble factors, such as prostaglandin E2, IDO, TGF-β, and human leukocyte antigen G5, and interactions between MSCs and immune cells such as T cells, B cells, macrophages, and dendritic cells.[69] SHED has significant effects on inhibiting T helper 17 cells compared to BMMSCs. SHED transplantation was capable of effectively reversing systemic lupus erythematosus (SLE)-associated disorders in SLE-like mice.[14] DPSCs could inhibit acute allogeneic immune responses by the release of TGF-β as a result of allogeneic stimulation of T lymphocytes.[70]
Systemic transplantation of SHED and DPSC in autoimmune disease mice model including SLE and inflammatory bowel disease ameliorated the tissue damages induced by hypersensitive immune response.[14,17,18]
Bone diseases
Systemic transplantation of mesenchymal stem cells could ameliorate bone loss and autoimmune disorders in a MRL/lpr mouse SLE mode by suppression of Interleukin-17 and maintaining a regular positive bone metabolism.[11] Systemic transplantation of SHED through the tail vein ameliorates ovariectomy (OVX)-induced osteopenia by reducing T-helper 1 and T-helper 17 cell numbers in the recipient OVX mice.[71]
Liver diseases
Terai et al.[72] administered bone marrow cells derived from GFP-labeled mice to carbon tetrachloride (CCl4)-induced liver injury model mice and found that these bone marrow cells engrafted in the injured liver, resulting in the absorption of fibrosis and the improvement of prognosis. The tooth germ progenitor cells prevented the progression of liver fibrosis in the liver of CCl4-treated rats and contributed to the restoration of liver function, as assessed by the measurement of hepatic serum markers aspartate aminotransferase and alanine aminotransferase.[12] Engraftment of DPSCs and SHED morphologically and functionally ameliorate acute and chronic injury of livers in CCl4-treated rats.[73]
Muscular
DPSCs can differentiate into dystrophin-producing multinucleated muscle cells and can be utilized in disorders such as muscular dystrophy, wherein, the body is unable to produce dystrophin. Utilization of myogenic progenitor cells derived from dental pulp produced more dystrophin as compared to the heterogeneously present stem cells.[74] Thereby proving to be a potential alternative for stem cell therapy in muscular dystrophy patients. Kerkis et al. used human DPSCs for the treatment of muscular dystrophy in golden retriever dogs, transplanted by arterial or muscular injections.[75]
Diabetes
Diabetes is a chronic degenerative disease. One of the treatments for diabetes includes transplantation of pancreatic islet cells. Chen et al. demonstrated that insulin-producing cells can be derived from monoclonal and polyclonal DPSCs.[76] Govindasamy et al. demonstrated that DPSCs have the capacity to differentiate into islet-like aggregates.[77]
Infertility
The potential of DPSCs can also be used in the treatment of infertility. Leake and Templeton isolated HDPSCs and injected them into the testes of live male mice. The mice were killed at various intervals after the injection, and their testes were examined to see whether the stem cells survived. It was found that stem cells settled in the testes and also differentiated into cells that were producing viable sperm.[78]
Cell bank for human dental pulp-derived stem cells
SHED isolated from the cryopreserved deciduous pulp tissues for over 2 years (SHED-Cryo) owned similar stem cell properties including clonogenicity, self-renew, stem cell marker expression, multipotency, in vivo tissue regenerative capacity and in vitro immunomodulatory function to SHED isolated from the fresh tissues (SHED-Fresh).[6] Induced pluripotent stem cells are constructed from dental pulp- derived stem cells and hold a great promise for regenerative medicine and other aspects of clinical applications.[79]
CONCLUSION
HDPSCs are useful in the treatment of various diseases as shown in this article. They have great potential and is a very powerful tool in regenerative medicine. They can be obtained safely and easily without significant morbidity or ethical concerns; however, the challenge of understanding the mechanisms underlying the therapeutic effects of DPSCs requires more research. The future treatment modality will be regenerative based; however, further studies are needed to test the various other applications of DPSCs with long-term follow-up.
Financial support and sponsorship
Nil.
Conflicts of interest
There are no conflicts of interest.
References
- Stem cell properties of human dental pulp stem cells. J Dent Res. 2002;81:531-5.
- [CrossRef] [PubMed] [Google Scholar]
- Postnatal human dental pulp stem cells (DPSCs) in vitro and in vivo. Proc Natl Acad Sci U S A. 2000;97:13625-30.
- [CrossRef] [PubMed] [Google Scholar]
- Properties and possibilities of human dental pulp-derived stem cells. Arch Stem Cell Res. 2015;2:1012.
- [Google Scholar]
- Dental pulp stem cells: Function, isolation and applications in regenerative medicine. J Tissue Eng Regen Med. 2015;9:1205-16.
- [CrossRef] [PubMed] [Google Scholar]
- Clinical potential and current progress of dental pulp stem cells for various systemic diseases in regenerative medicine: A concise review. Int J Mol Sci. 2019;20:e1132.
- [CrossRef] [PubMed] [Google Scholar]
- Cryopreserved dental pulp tissues of exfoliated deciduous teeth is a feasible stem cell resource for regenerative medicine. PLoS One. 2012;7:e51777.
- [CrossRef] [PubMed] [Google Scholar]
- Dental stem cells and their sources. Dent Clin North Am. 2012;56:549-61.
- [CrossRef] [PubMed] [Google Scholar]
- SHED: Stem cells from human exfoliated deciduous teeth. Proc Natl Acad Sci U S A. 2003;100:5807-12.
- [CrossRef] [PubMed] [Google Scholar]
- Immune therapeutic potential of stem cells from human supernumerary teeth. J Dent Res. 2013;92:609-15.
- [CrossRef] [PubMed] [Google Scholar]
- Mesenchymal stem cell-mediated functional tooth regeneration in swine. PLoS One. 2006;1:e79.
- [CrossRef] [PubMed] [Google Scholar]
- Transplantation of mesenchymal stem cells ameliorates secondary osteoporosis through interleukin-17-impaired functions of recipient bone marrow mesenchymal stem cells in MRL/lpr mice. Stem Cell Res Ther. 2015;6:104.
- [CrossRef] [PubMed] [Google Scholar]
- Multipotent cells from the human third molar: Feasibility of cell-based therapy for liver disease. Differentiation. 2008;76:495-505.
- [CrossRef] [PubMed] [Google Scholar]
- Transplantation of islet-like cell clusters derived from human dental pulp stem cells restores normoglycemia in diabetic mice. Cytotherapy. 2013;15:1228-36.
- [CrossRef] [PubMed] [Google Scholar]
- Immunomodulatory properties of stem cells from human exfoliated deciduous teeth. Stem Cell Res Ther. 2010;1:5.
- [CrossRef] [PubMed] [Google Scholar]
- Immunomodulatory properties of human periodontal ligament stem cells. J Cell Physiol. 2009;219:667-76.
- [CrossRef] [PubMed] [Google Scholar]
- Effect of cryopreservation on biological and immunological properties of stem cells from apical papilla. J Cell Physiol. 2010;223:415-22.
- [CrossRef] [PubMed] [Google Scholar]
- Fas ligand regulates the immunomodulatory properties of dental pulp stem cells. J Dent Res. 2012;91:948-54.
- [CrossRef] [PubMed] [Google Scholar]
- Acetylsalicylic acid treatment improves differentiation and immunomodulation of SHED. J Dent Res. 2015;94:209-18.
- [CrossRef] [PubMed] [Google Scholar]
- Pulp regeneration in a full-length human tooth root using a hierarchical nanofibrous microsphere system. Acta Biomater. 2016;35:57-67.
- [CrossRef] [PubMed] [Google Scholar]
- Nanofibrous spongy microspheres for the delivery of hypoxia-primed human dental pulp stem cells to regenerate vascularized dental pulp. Acta Biomater. 2016;33:225-34.
- [CrossRef] [PubMed] [Google Scholar]
- Stem/progenitor cell-mediated de novo regeneration of dental pulp with newly deposited continuous layer of dentin in an in vivo model. Tissue Eng Part A. 2010;16:605-15.
- [CrossRef] [PubMed] [Google Scholar]
- Dentin regeneration by dental pulp stem cell therapy with recombinant human bone morphogenetic protein 2. J Dent Res. 2004;83:590-5.
- [CrossRef] [PubMed] [Google Scholar]
- Enhancement of periodontal tissue regeneration by transplantation of bone marrow mesenchymal stem cells. J Periodontol. 2004;75:1281-7.
- [CrossRef] [PubMed] [Google Scholar]
- Stem cell therapy in oral and maxillofacial region: An overview. J Oral Maxillofac Pathol. 2012;16:58-63.
- [CrossRef] [PubMed] [Google Scholar]
- Experimental formation of periodontal structure around titanium implants utilizing bone marrow mesenchymal stem cells: A pilot study. J Oral Implantol. 2009;35:106-29.
- [CrossRef] [PubMed] [Google Scholar]
- In situ tissue engineering of periodontal tissues by seeding with periodontal ligament-derived cells. Tissue Eng. 2004;10:537-44.
- [CrossRef] [PubMed] [Google Scholar]
- Periodontal regeneration with multi-layered periodontal ligament-derived cell sheets in a canine model. Biomaterials. 2009;30:2716-23.
- [CrossRef] [PubMed] [Google Scholar]
- Periodontal ligament stem cell-mediated treatment for periodontitis in miniature swine. Stem Cells. 2008;26:1065-73.
- [CrossRef] [PubMed] [Google Scholar]
- Regenerative potential of human periodontal ligament derived stem cells on three-dimensional biomaterials: A morphological report. J Biomed Mater Res A. 2008;87:986-93.
- [CrossRef] [PubMed] [Google Scholar]
- Bone regeneration by human dental pulp stem cells using a helioxanthin derivative and cell-sheet technology. Stem Cell Res Ther. 2018;9:24.
- [CrossRef] [PubMed] [Google Scholar]
- Bone healing by sterilizable calcium phosphate tetrapods eluting osteogenic molecules. Biomaterials. 2013;34:5530-7.
- [CrossRef] [PubMed] [Google Scholar]
- Enhancement of bone formation ex vivo and in vivo by a helioxanthin-derivative. Biochem Biophys Res Commun. 2010;395:502-8.
- [CrossRef] [PubMed] [Google Scholar]
- A novel osteogenic helioxanthin-derivative acts in a BMP-dependent manner. Biochem Biophys Res Commun. 2007;357:854-60.
- [CrossRef] [PubMed] [Google Scholar]
- Human mandible bone defect repair by the grafting of dental pulp stem/progenitor cells and collagen sponge biocomplexes. Eur Cell Mater. 2009;18:75-83.
- [CrossRef] [PubMed] [Google Scholar]
- Reconstruction of large cranial defects in nonimmunosuppressed experimental design with human dental pulp stem cells. J Craniofac Surg. 2008;19:204-10.
- [CrossRef] [PubMed] [Google Scholar]
- Osteogenic differentiation of stem cells derived from human periodontal ligaments and pulp of human exfoliated deciduous teeth. Cell Tissue Res. 2010;340:323-33.
- [CrossRef] [PubMed] [Google Scholar]
- Dental stem cells and their applications in dental tissue engineering. Open Dent J. 2013;7:76-81.
- [CrossRef] [PubMed] [Google Scholar]
- Bioengineered teeth from cultured rat tooth bud cells. J Dent Res. 2004;83:523-8.
- [CrossRef] [PubMed] [Google Scholar]
- Histological and immunohistochemical studies of tissue engineered odontogenesis. Arch Histol Cytol. 2005;68:89-101.
- [CrossRef] [PubMed] [Google Scholar]
- Mesenchymal stem cells of dental origin as promising tools for neuroregeneration. Stem Cell Res Ther. 2014;5:61.
- [CrossRef] [PubMed] [Google Scholar]
- Human dental pulp-derived stem cells promote locomotor recovery after complete transection of the rat spinal cord by multiple neuro-regenerative mechanisms. J Clin Invest. 2012;122:80-90.
- [CrossRef] [PubMed] [Google Scholar]
- Human dental pulp cells differentiate toward neuronal cells and promote neuroregeneration in adult organotypic hippocampal slices in vitro. Int J Mol Sci. 2017;18:e1745.
- [CrossRef] [PubMed] [Google Scholar]
- Chrysin suppressed inflammatory responses and the inducible nitric oxide synthase pathway after spinal cord injury in rats. Int J Mol Sci. 2014;15:12270-9.
- [CrossRef] [PubMed] [Google Scholar]
- Potential roles of dental pulp stem cells in neural regeneration and repair. Stem Cells Int. 2018;2018:1731289.
- [CrossRef] [PubMed] [Google Scholar]
- Multifaceted neuro-regenerative activities of human dental pulp stem cells for functional recovery after spinal cord injury. Neurosci Res. 2014;78:16-20.
- [CrossRef] [PubMed] [Google Scholar]
- Analysis of the neuroregenerative activities of mesenchymal stem cells in functional recovery after rat spinal cord injury. Methods Mol Biol. 2014;1213:321-8.
- [CrossRef] [PubMed] [Google Scholar]
- Potential of human dental stem cells in repairing the complete transection of rat spinal cord. J Neural Eng. 2017;14:26005.
- [CrossRef] [PubMed] [Google Scholar]
- Taking a bite out of spinal cord injury: Do dental stem cells have the teeth for it? Cell Mol Life Sci. 2016;73:1413-37.
- [CrossRef] [PubMed] [Google Scholar]
- Chitosan scaffolds induce human dental pulp stem cells to neural differentiation: Potential roles for spinal cord injury therapy. Cell Tissue Res. 2016;366:129-42.
- [CrossRef] [PubMed] [Google Scholar]
- Dental pulp-derived CD31¯/CD146¯ side population stem/progenitor cells enhance recovery of focal cerebral ischemia in rats. Tissue Eng Part A. 2011;17:1303-11.
- [CrossRef] [PubMed] [Google Scholar]
- A simple and efficient method for generating nurr1-positive neuronal stem cells from human wisdom teeth (tNSC) and the potential of tNSC for stroke therapy. Cytotherapy. 2009;11:606-17.
- [CrossRef] [PubMed] [Google Scholar]
- Human adult dental pulp stem cells enhance poststroke functional recovery through non-neural replacement mechanisms. Stem Cells Transl Med. 2012;1:177-87.
- [CrossRef] [PubMed] [Google Scholar]
- Concise review: Preclinical studies on human cell-based therapy in rodent ischemic stroke models: Where are we now after a decade? Stem Cells. 2013;31:1040-3.
- [CrossRef] [PubMed] [Google Scholar]
- Comparison of the effects of human dental pulp stem cells and human bone marrow-derived mesenchymal stem cells on ischemic human astrocytes in vitro. J Neurosci Res. 2015;93:973-83.
- [CrossRef] [PubMed] [Google Scholar]
- Effect of dental pulp stem cells in MPTP-induced old-aged mice model. Eur J Clin Invest. 2017;47:403-14.
- [CrossRef] [PubMed] [Google Scholar]
- Neuroimmunomodulatory properties of DPSCs in an in vitro model of Parkinson's disease. IUBMB Life. 2017;69:689-99.
- [CrossRef] [PubMed] [Google Scholar]
- Alzheimer's disease: Strategies for disease modification. Nat Rev Drug Discov. 2010;9:387-98.
- [CrossRef] [PubMed] [Google Scholar]
- Alzheimer mechanisms and therapeutic strategies. Cell. 2012;148:1204-22.
- [CrossRef] [PubMed] [Google Scholar]
- Dental pulp stem cells promote regeneration of damaged neuron cells on the cellular model of Alzheimer's disease. Cell Biol Int. 2017;41:639-50.
- [CrossRef] [PubMed] [Google Scholar]
- Therapeutic potential of dental pulp stem cell secretome for Alzheimer's disease treatment: An in vitro study. Stem Cells Int. 2016;2016:8102478.
- [CrossRef] [PubMed] [Google Scholar]
- Conditioned medium from the stem cells of human dental pulp improves cognitive function in a mouse model of Alzheimer's disease. Behav Brain Res. 2015;293:189-97.
- [CrossRef] [PubMed] [Google Scholar]
- Autophagy in axonal degeneration in glaucomatous optic neuropathy. Prog Retin Eye Res. 2015;47:1-8.
- [CrossRef] [PubMed] [Google Scholar]
- Intravitreally transplanted dental pulp stem cells promote neuroprotection and axon regeneration of retinal ganglion cells after optic nerve injury. Invest Ophthalmol Vis Sci. 2013;54:7544-56.
- [CrossRef] [PubMed] [Google Scholar]
- Mesenchymal stromal cell-mediated neuroprotection and functional preservation of retinal ganglion cells in a rodent model of glaucoma. Cytotherapy. 2016;18:487-96.
- [CrossRef] [PubMed] [Google Scholar]
- PLGA artificial nerve conduits with dental pulp cells promote facial nerve regeneration. J Tissue Eng Regen Med. 2011;5:823-30.
- [CrossRef] [PubMed] [Google Scholar]
- Engineered neural tissue with Schwann cell differentiated human dental pulp stem cells: Potential for peripheral nerve repair? J Tissue Eng Regen Med. 2017;11:3362-72.
- [CrossRef] [PubMed] [Google Scholar]
- Immunomodulatory properties of dental tissue-derived mesenchymal stem cells. Oral Dis. 2014;20:25-34.
- [CrossRef] [PubMed] [Google Scholar]
- Human dental pulp stem cells suppress alloantigen-induced immunity by stimulating T cells to release transforming growth factor beta. J Endod. 2017;43:100-8.
- [CrossRef] [PubMed] [Google Scholar]
- Transplantation of SHED prevents bone loss in the early phase of ovariectomy-induced osteoporosis. J Dent Res. 2014;93:1124-32.
- [CrossRef] [PubMed] [Google Scholar]
- An in vivo model for monitoring trans-differentiation of bone marrow cells into functional hepatocytes. J Biochem. 2003;134:551-8.
- [CrossRef] [PubMed] [Google Scholar]
- Novel management of acute or secondary biliary liver conditions using hepatically differentiated human dental pulp cells. Tissue Eng Part A. 2015;21:586-93.
- [CrossRef] [PubMed] [Google Scholar]
- Clones of ectopic stem cells in the regeneration of muscle defects in vivo. PLoS One. 2010;5:e13547.
- [CrossRef] [PubMed] [Google Scholar]
- Early transplantation of human immature dental pulp stem cells from baby teeth to golden retriever muscular dystrophy (GRMD) dogs: Local or systemic? J Transl Med. 2008;6:35.
- [CrossRef] [PubMed] [Google Scholar]
- Insulin-Producing Cells (IPCS) from Dental-pulp Stem/Progenitor Cells. Available from: http://www.stemsave.com/Diabetes.aspx (accessed )
- [Google Scholar]
- Differentiation of dental pulp stem cells into islet-like aggregates. J Dent Res. 2011;90:646-52.
- [CrossRef] [PubMed] [Google Scholar]
- Mice Produce Human Sperm to Raise Hope for Infertile Men. 2008. The Sunday Times. Available from: http://www.thesundaytimes.co.uk (accessed )
- [Google Scholar]
- IPS cells reprogrammed from human mesenchymal-like stem/progenitor cells of dental tissue origin. Stem Cells Dev. 2010;19:469-80.
- [CrossRef] [PubMed] [Google Scholar]